Mount Everest Is Still Growing
You might think Mount Everest has reached its maximum height, but Earth's highest peak won't stop growing anytime soon. At about 2 millimeters per year, this giant continues to stretch skyward through a complex dance of tectonic forces and natural processes. While this growth may seem minimal, it represents an active geological marvel that's been ongoing for millions of years. The story behind this persistent rise reveals fascinating insights into our planet's dynamic nature.
The Dynamic Nature of Earth's Tallest Peak
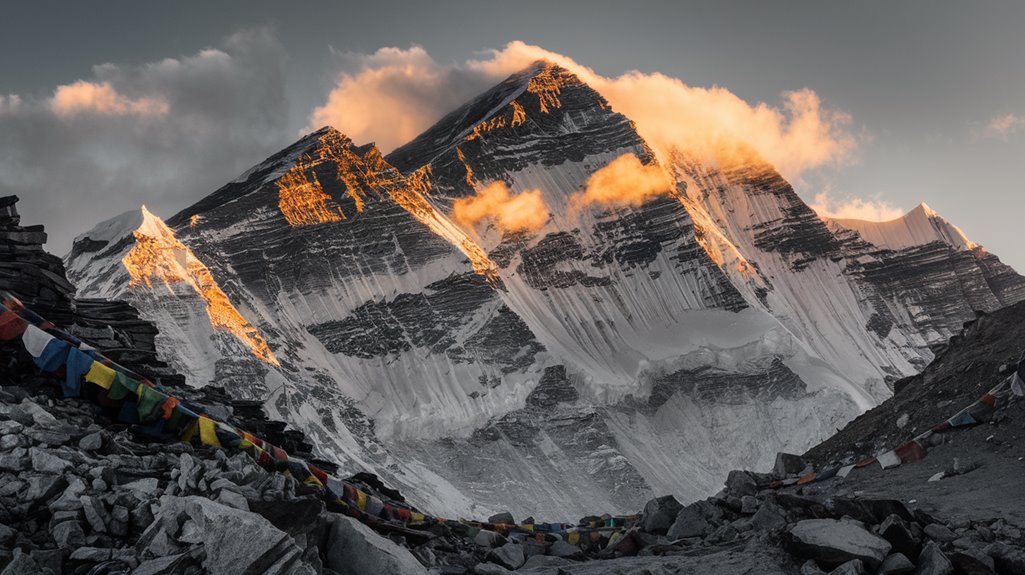
While many people think of Mount Everest as a static monument of nature, it's actually growing taller each year through a fascinating combination of geological processes.
You'll find that Earth's highest peak gains about 2 millimeters annually – twice what scientists previously believed. This growth stems from the dynamic interplay between tectonic forces, river erosion, and a process called isostatic rebound. The unique interaction between erosive power and mantle pressure creates a continuous feedback loop that sustains the mountain's growth.
The formation of this majestic peak began when the Indian and Eurasian plates collided approximately 50 million years ago.
Despite climate impacts affecting the region's glaciers, Everest demonstrates remarkable geological resilience.
The mountain's growth is primarily driven by the erosive forces of the Arun and Kosi rivers, which carve away material from the surrounding landscape. As this weight lifts from the Earth's crust, the mountain responds by rising upward, showcasing how even the world's mightiest peaks are in constant transformation.
Understanding Isostatic Rebound
To fully understand Mount Everest's growth, you'll need to grasp the concept of isostatic rebound, a remarkable process where Earth's crust rises after being relieved of heavy loads.
Think of it like a compressed sponge returning to its original shape – when weight is removed from Earth's surface, the crust naturally bounces back.
These isostatic effects can considerably impact mountain heights, contributing up to 25% of peak elevations in temperate regions.
The process occurs as Earth's asthenosphere, which behaves like a fluid, responds to changes in pressure above it.
When glaciers melt or erosion removes material, crustal uplift follows.
Understanding this process follows a clear structure-function sequence as we examine how the Earth's layers interact and respond to pressure changes.
You can observe this phenomenon today in places like Canada, where the land is still rising from the weight of ice sheets that melted thousands of years ago.
In polar climates, this process can be even more dramatic, with glacial erosion generating significantly more relief and peak uplift than in temperate regions.
Rivers and Their Role in Mountain Growth
Rivers play a surprising role in Mount Everest's growth story. When the Arun River underwent a dramatic event called river capture about 89,000 years ago, it merged with another river system in Tibet, considerably boosting its erosive power. This merger changed the region's erosion patterns dramatically. The Arun River's location 75 kilometers from Everest makes its erosive effects particularly significant.
The ongoing height increase has been confirmed through GPS monitoring systems that show the mountain rising approximately two millimeters annually.
You'll find it fascinating that as rivers like the Arun and Kosi carve deep gorges and remove billions of tons of earth, they're actually helping Everest grow taller.
Through a process called isostatic rebound, the removal of material by these rivers reduces pressure on the Earth's crust, causing it to rise. While tectonic forces remain the primary driver of Everest's growth, the rivers' erosive action has contributed between 15 to 50 meters to its height over 89,000 years.
Modern Measurement Technologies
Since measuring Earth's highest peak demands exceptional precision, scientists now employ an impressive array of modern technologies to track Mount Everest's exact height.
You'll find that gravity measurements from aircraft, combined with GPS surveys, provide unprecedented accuracy in determining the mountain's true elevation.
Modern measurement techniques include:
- Ground penetrating radar to measure snow depth at the peak
- Aircraft-mounted gravimeters to assess gravitational pull
- GPS antennas for precise positioning data
- Advanced computational methods to process complex measurements
These technologies have helped establish Everest's current height at 8848.86 meters.
What's particularly fascinating is that you can now distinguish between the actual rock height and the snow cover above it, thanks to sophisticated radar equipment that performs reliably even in extreme conditions.
Similar to how real-time data collection helps assess changes in consumer behavior, these measurement tools provide continuous monitoring of the mountain's growth.
A King Air turboprop aircraft was specifically used to conduct gravity measurements by flying parallel lines over the mountain.
Historical Formation and Tectonic Forces
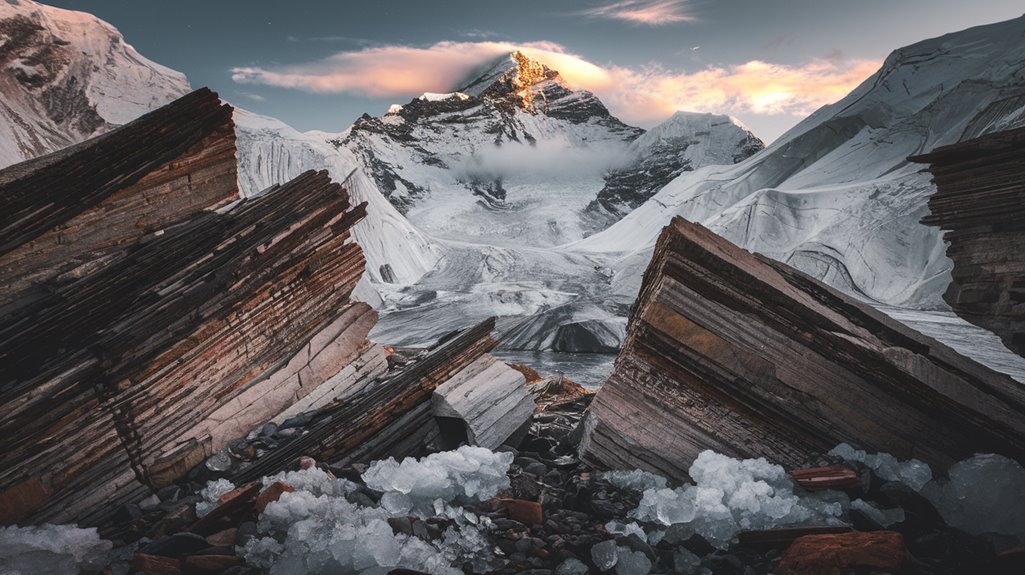
While Earth's tallest peak continues to grow today, Mount Everest's dramatic formation began roughly 60 million years ago when the Indian tectonic plate collided with the Eurasian plate.
Through a series of tectonic collisions, the limestone, sandstone, and shale of the Indian plate's seabed were thrust upward, creating the massive Himalayan range.
These geological processes involved complex transformations as rock layers stacked along thrust faults, with significant metamorphism occurring at depths beyond 15 kilometers.
You'll find evidence of this intense activity in the mountain's structure, where marine sediments from India's continental shelf now sit atop the Rongbuk Formation's metamorphic and granitic rocks.
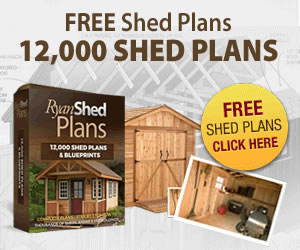
The mountain's composition reflects its marine origins, with the Qomolangma Formation consisting primarily of limestone and dolomite from ancient seafloor deposits.